Would planes be better if they were more like birds?
Eagle-inspired engineering
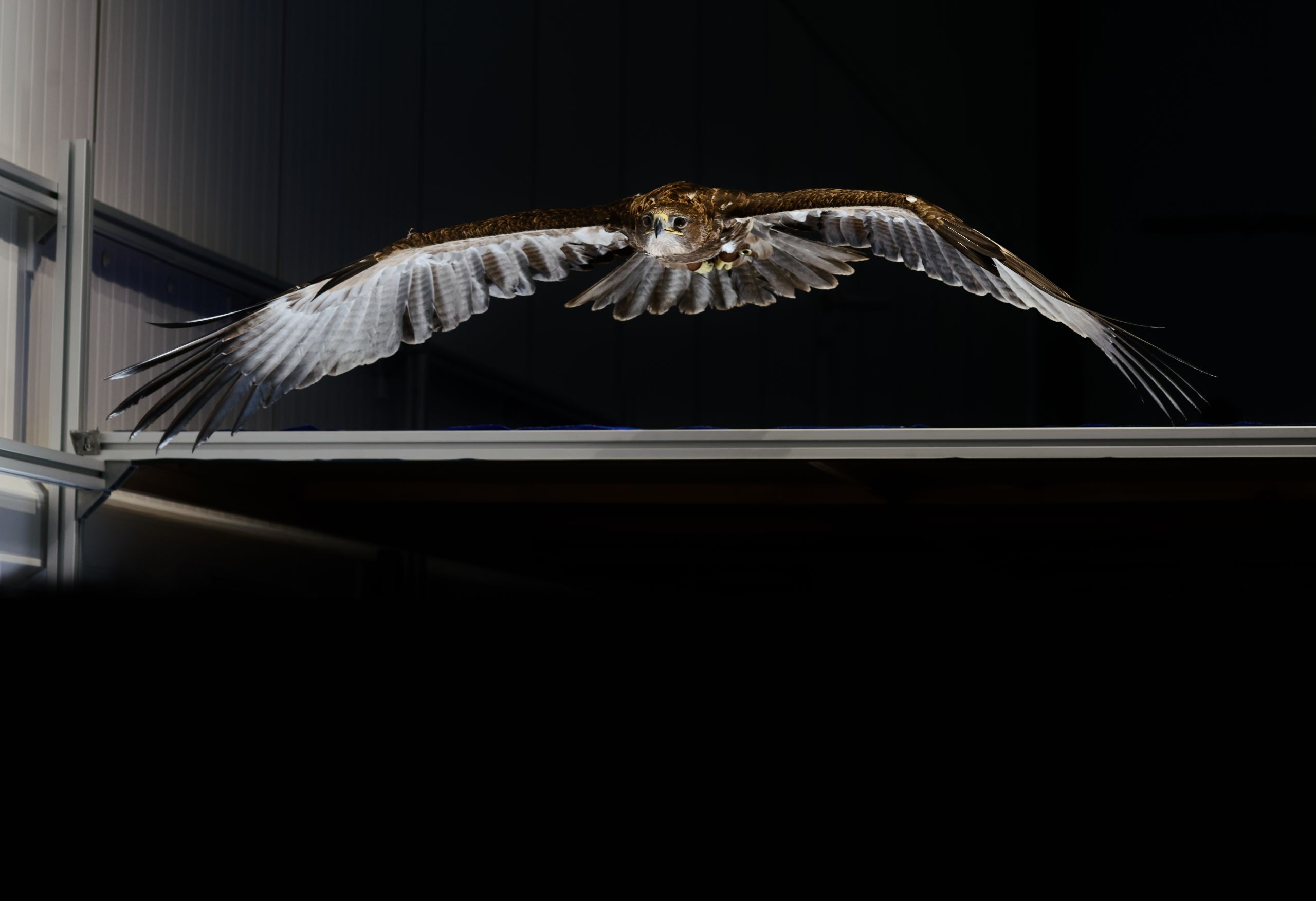
Is it a bird..? Is it a plane..? Silhouetted against the sky, sometimes it is hard to tell. Let us compare their features; birds and most aeroplanes have two wings attached to the main body and a tail at the back.
The similarities between these two sky-users – one natural, one manmade – are not coincidental. The engineering marvel of air travel is built on biological inspiration. After an eternity dreaming of flight, it was logical to look to nature’s successful examples when designing flying machines.
But, after we have observed that our blueprint is to attach two wings and a tail to the body, that is pretty much where our similarities end. Would planes be better if they were more like birds?
At the Royal Veterinary College and University of Bristol, we turned to our feathered friends and discovered two ways they might inspire aircraft of the future..
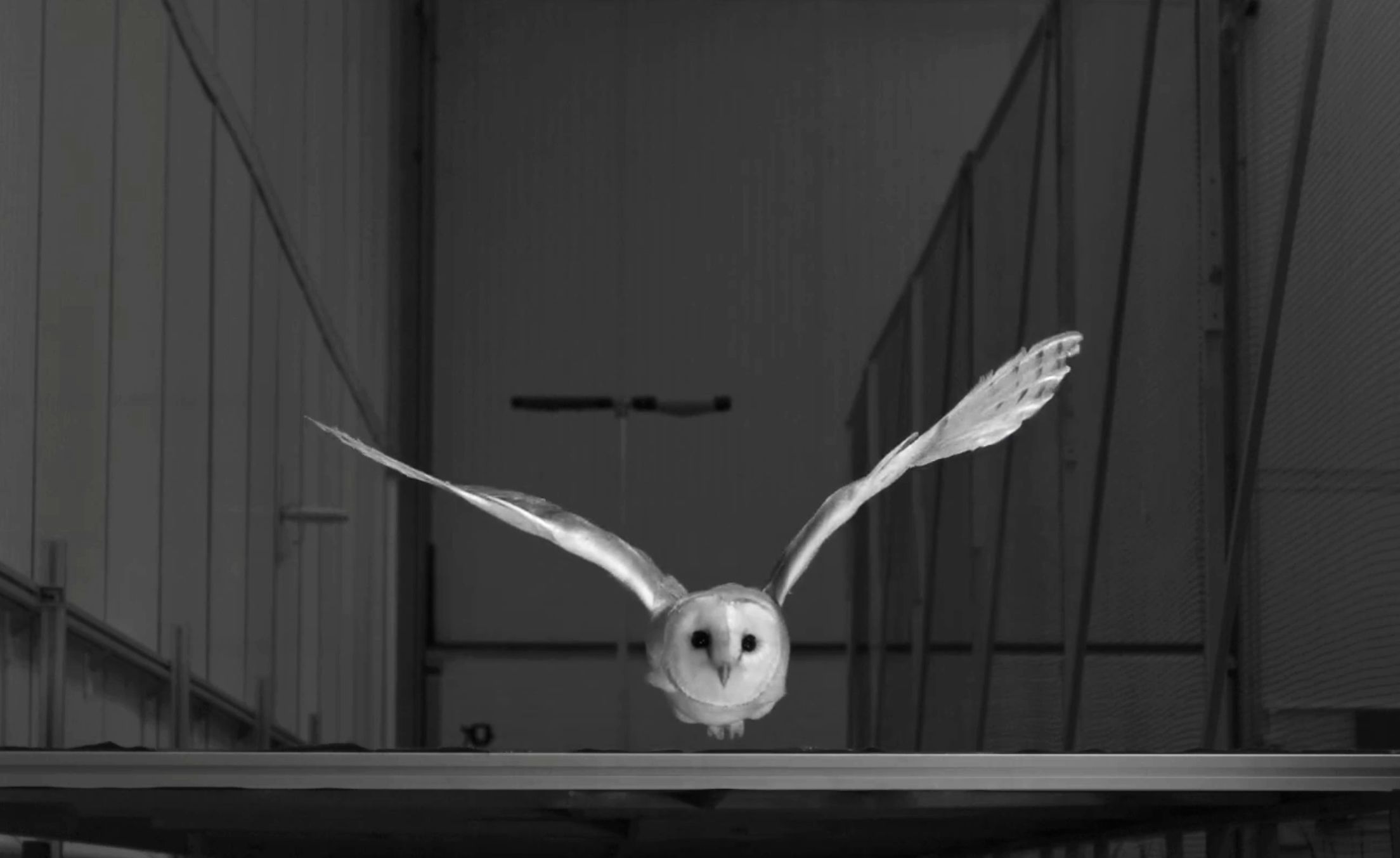
Lily the barn owl flies through a wind gust that would challenge an aeroplane of similar size.
Lily the barn owl flies through a wind gust that would challenge an aeroplane of similar size.
The first story highlights how we might avoid the unpleasant jolts experienced as aircraft fly through turbulence by attaching the wings to a hinged suspension system.
Discover how birds deal with strong wind gusts by playing our game It’s a Breeze. Then use Augmented Reality on your phone to invite Lily the barn owl to glide through your own home or outside space with OwlAR.
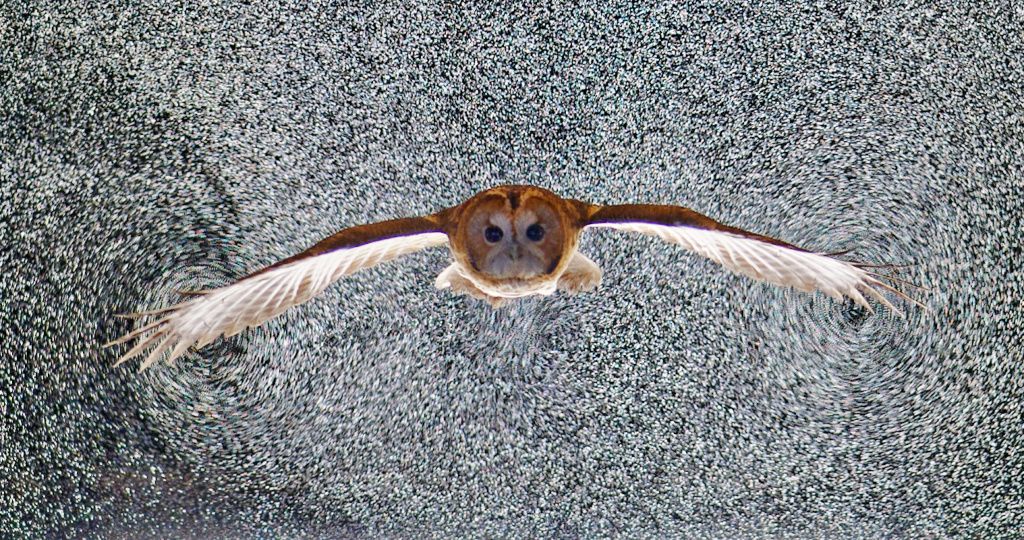
Hector the tawny owl glides through a mass of bubbles that reveal how his tail uses the air to produce aerodynamic force.
Hector the tawny owl glides through a mass of bubbles that reveal how his tail uses the air to produce aerodynamic force.
The second story tells of how bird-sized vehicles, that may soon be delivering parcels to your house or medicines to remote areas, can save on fuel (or increase range and battery life) by emulating the way birds use their tails.
Dealing with turbulence
Have you ever flown through turbulence? The pilot might have said, “Fasten your seatbelts, everyone; it’s going to be a bumpy ride”, or perhaps something more soothing…
Birds can fly in gusty conditions that our best aircraft of that scale never manage. This is an important capability for birds because they simply cannot afford to risk serious injury by missing that perch on a windy cliffside, or failing too many attempts to catch prey when out hunting.
Play our game It’s a Breeze to get started with the physics of flight through gusts.
Making an artificial gust
Is there something birds can teach us about coping with turbulence? We thought there probably was, so we invited Lily the barn owl, Sasha the tawny eagle, Ellie the goshawk and some of their friends to fly through gusts we made in our laboratory.
Inside our purpose-built flight lab, we created an artificial gust by building a wind tunnel on its side, so that the blowing end was pointing at the ceiling. The birds flew down the track, began to glide on the approach to landing and then – whoosh – they experienced a strong gust of wind from below. Well, it didn’t start that way. First, we had the fans running very slowly so the birds almost didn’t notice the gust—it was just a gentle breeze. As we all grew in confidence, we turned up the fans so the gust almost equalled the birds’ forward speed. For the birds, it was no problem at all. They were barely knocked off course. How did they do it?
Birds wings act like a suspension system
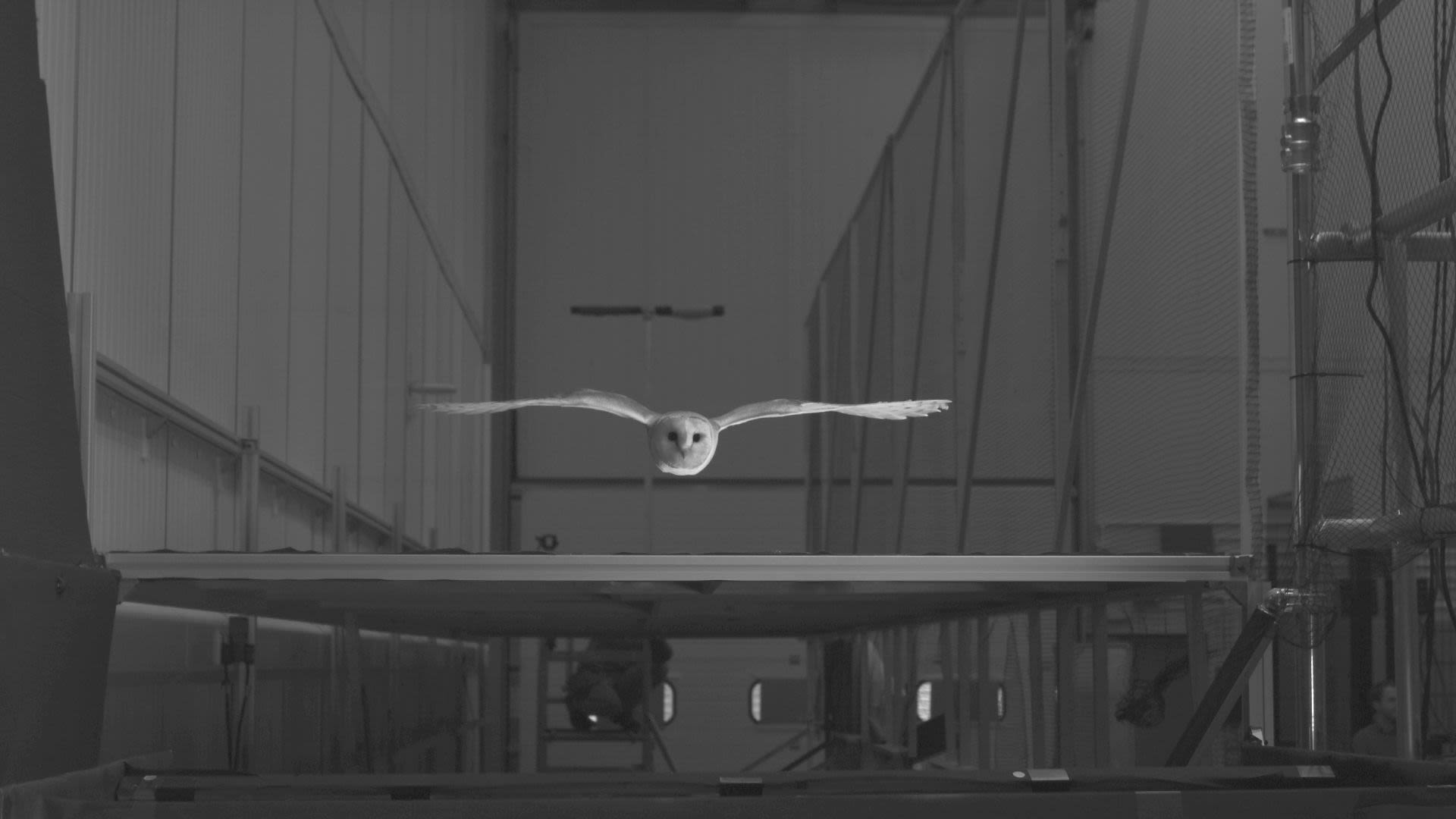
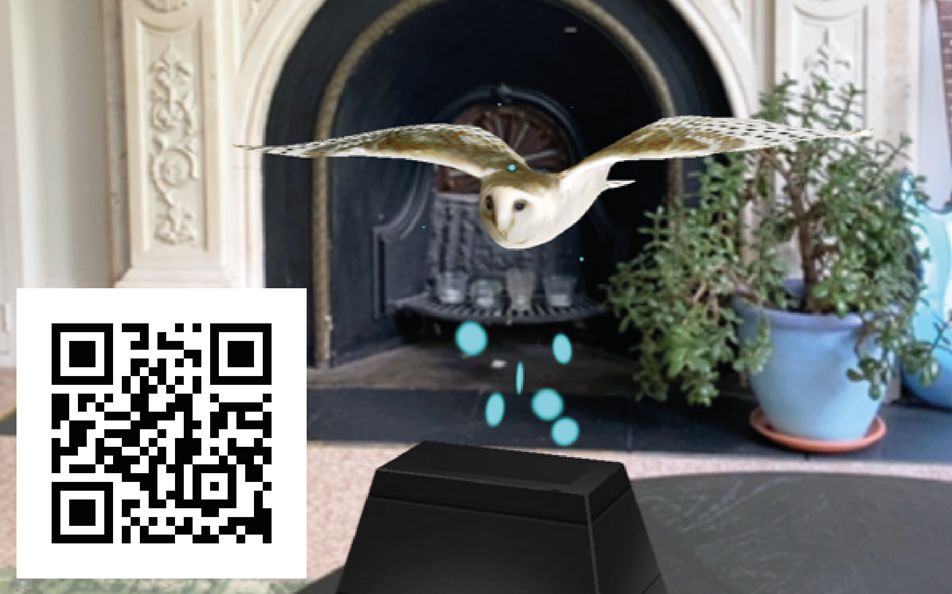
Recreate our experiment in your own home using Augmented Reality with OwlAR! Scan this QR code with your phone to see Lily gliding through gusts for fun. If you are reading on a phone or tablet, you can click here. Freeze the flight at any time to study the position of her wings.
Recreate our experiment in your own home using Augmented Reality with OwlAR! Scan this QR code with your phone to see Lily gliding through gusts for fun. If you are reading on a phone or tablet, you can click here. Freeze the flight at any time to study the position of her wings.
Bird wings have a sweet spot
Did you notice how Lily’s wings elevate rapidly while her head and body continue on a steady path? There is an elegant physical explanation for this, and it all boils down to two properties of birds’ wings. The first is the centre of pressure – the location where the gust pushes on each wing if you think of it as acting at a single point. The second is the centre of percussion. This will have a more familiar name to anyone who plays cricket, baseball, or other bat or racquet sports: the ‘sweet spot’. If you hit a ball close to the very far end of a bat, the handle jerks forward out of your hand. Conversely, if you hit the ball close to the handle, the handle jars backwards in your hand. This must mean that in between these impact locations there is a point where the handle neither jerks forwards nor jars backwards: this is the sweet spot. An impulse hitting here jolts the bat both in the direction of the impulse (translation) and around your hands (rotation). These two responses cancel each other at the handle, and you can hit a cricket ball, baseball, or golf ball a really long way without bruising your hands.
How does this relate to bird flight? Well, the impact of the ball is now the extra force applied by the gust at the centre of pressure, and the handle is the bird’s shoulder. If the gust force coincides with the centre of percussion, then the wing rotates around the shoulder joint without jolting the body up or down.
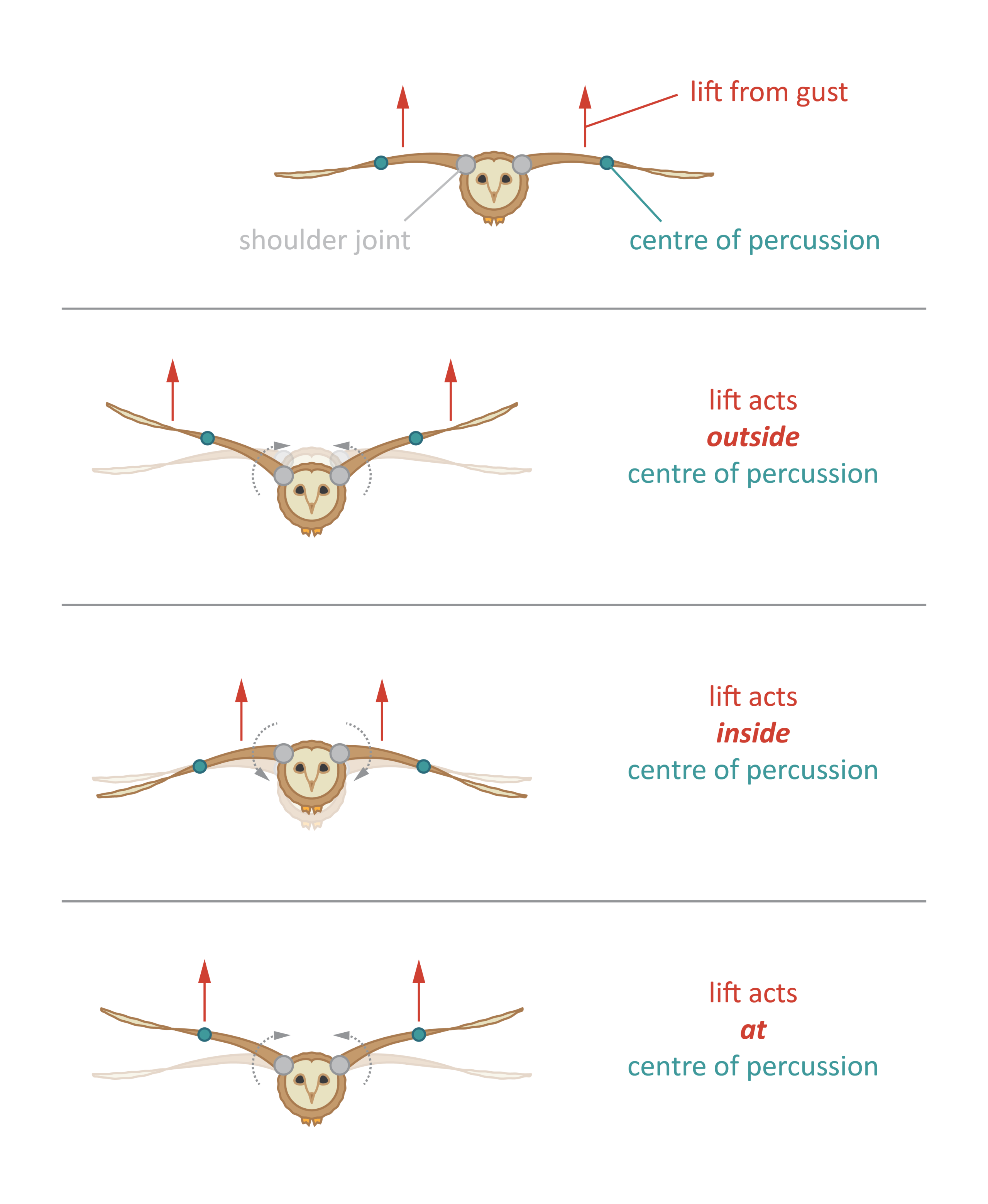
If the wings are set up in the right way, the gust moves them without disturbing the body
If the wings are set up in the right way, the gust moves them without disturbing the body
It is a suspension system, and it works by decoupling the mass of the wings from the mass of the body. The gust is permitted to accelerate the wings upwards but only in a way that will not transfer the translational force and rotational torque to the body of the bird. Similarly, car suspension is set up so that the forces and torques from bumps on the road don’t cause your body to bump about too much, even while the chassis gets jolted. Car design can even reach the extent of minimizing jolts experienced by the driver’s head, leaving the rest of the body to wobble a bit.
Now watch this video of Sasha the tawny eagle doing the same thing. Can you see his feathers ruffle as he enters the gust? Can you see how the wing elevates around the shoulder without his body getting knocked off course?
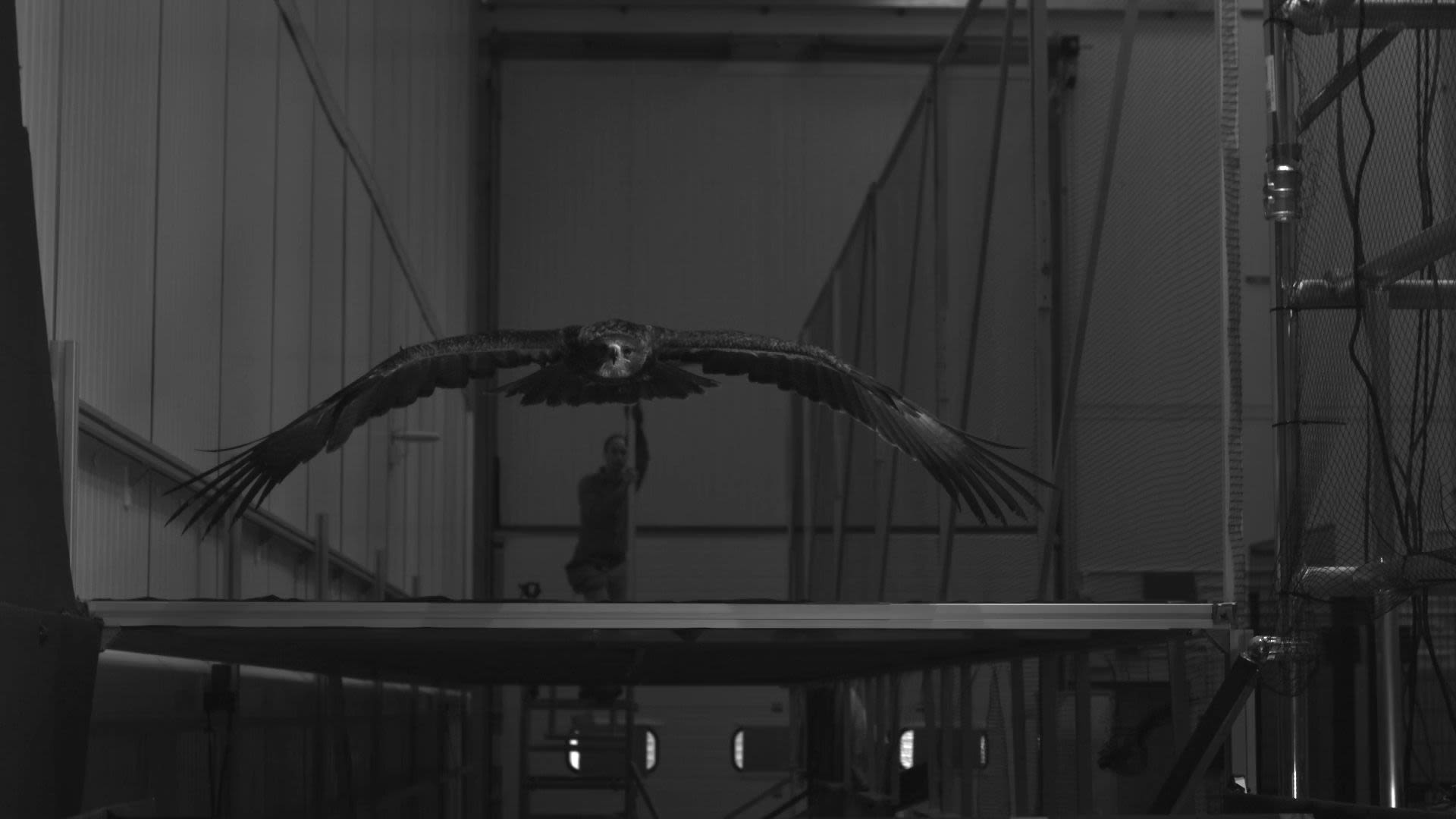
Exquisite, isn’t it? And all the more beautiful for knowing the physical principles underpinning how it works. Now all we have to do is transfer what we have learnt from the birds to aeroplanes.
Hinging wings reduce the effect of gusts
We built a prototype glider and flew it through the same gusty conditions. It was very important to give it the proper physical characteristics; not feathers, but specially designed hinging wings:
- The centre of pressure must align with the centre of percussion. We achieved this by adding nuts and bolts to just the right point along the wing;
- Each wing must be fixed to the fuselage by a hinge representing the bird’s shoulder joint, rather than fused rigidly;
- Each wing must be held in place in flight by a spring that supports Weight during flight but allows movement around the hinge if the aircraft enters a gust. (For the budding physicists: it turns out that the best kind of spring is an unusual one, where the force doesn’t change with length. Muscles, such as the pectoralis flight muscles of birds, are particularly good for achieving this property, but here we used long elastic bands!)
The glider on the right has gust-absorbing hinged wings and its body gets knocked upwards less violently
The glider on the right has gust-absorbing hinged wings and its body gets knocked upwards less violently
How do birds use their tails?
Introduction to flight forces
Birds and planes must obey the very same laws of physics, and a wing is a pretty good way to create the aerodynamic force known as Lift which balances the Weight of the animal, or aeroplane, due to the relentless pull of gravity. However, there are several notable differences between the two fliers. You may have noticed that aeroplanes don’t flap. Flapping is a way to reorient the wings and the aerodynamic force they produce to propel animals forwards in order to balance another force they are experiencing: Drag. In short, Drag slows you down and is the reason that paper aeroplanes don’t go on gliding forever. Planes take a simpler approach to overcoming Drag by using engines for thrust; that may be a jet engine, or a motor-driven propeller. Jets and propellers are relatively rare forms of biological locomotion and not used at all by animals to move through air.
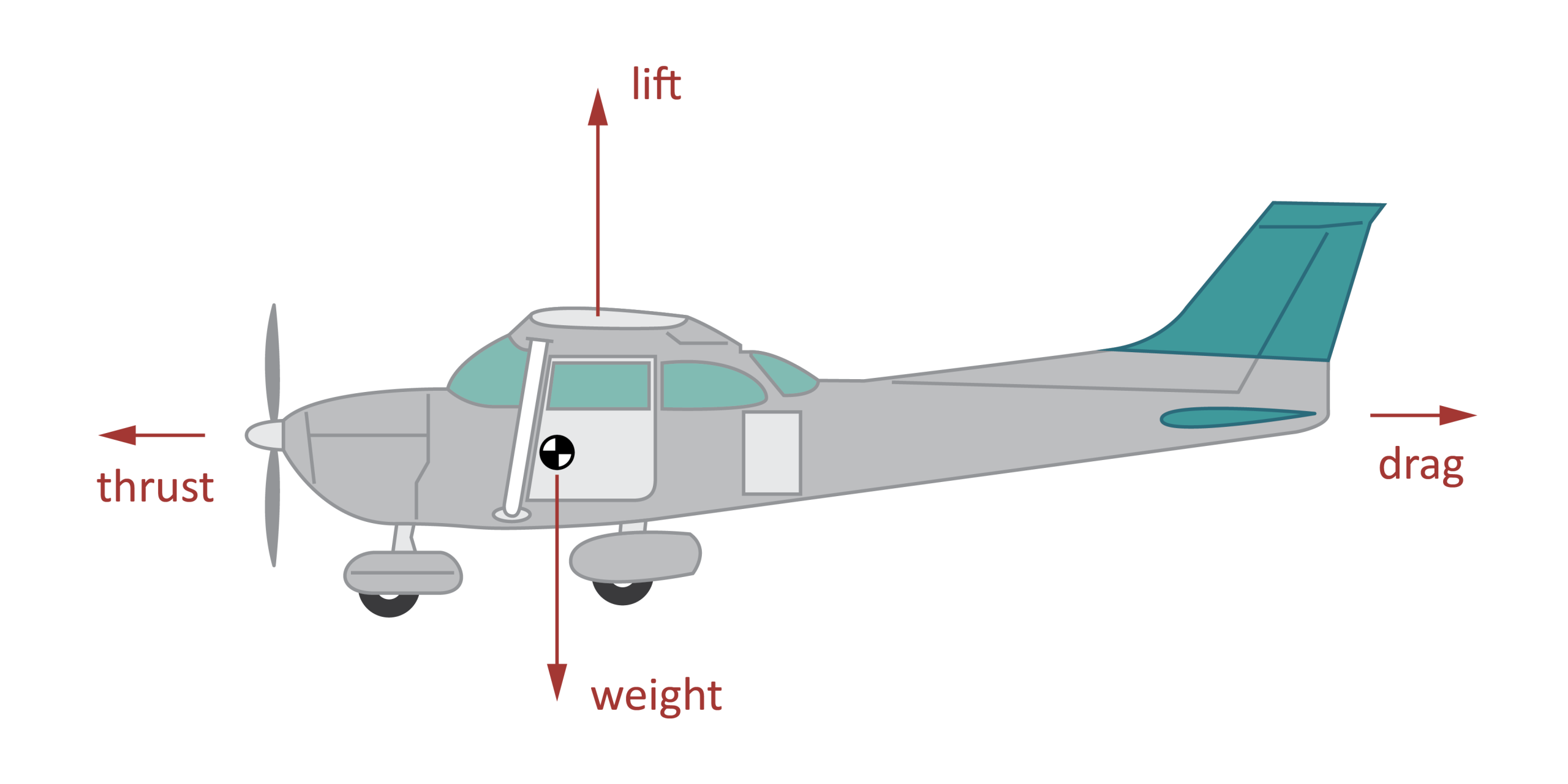
Balance of forces on an aircraft in steady level flight
Balance of forces on an aircraft in steady level flight
Wings produce the Lift that keeps animals and aeroplanes aloft against the force of Weight. Whether or not a wing is producing Lift it will always experience some Drag from the friction of air as it moves over the wing surfaces. When the wing does start producing Lift, however, Drag begins to rise. Aeroplane and racing car makers constantly search for ways to reduce this Drag while maintaining Lift.
Some cars use rear spoilers to produce downward ‘Lift’, known as Downforce. This helps the car stick tightly to the road when taking corners at high speed but is bad news for fuel economy. To maximise miles per gallon, your best bet is to slip through the air while disturbing it as little as possible – this is the concept of streamlining.
It might surprise you to read that, like racing cars, most passenger aeroplanes also produce Downforce with their tails. In practice, this severely impairs their flight economy because the wings have to produce even more Lift in compensation. Drag increases accordingly, and the engines have to work even harder. So why do aircraft designers set the tails at that angle?
The answer is stability. With the wings angled positively (generating Lift) and the tail angled negatively (generating Downforce), in normal ‘trimmed’ flight, the weight of the aircraft must be slightly ahead of the wings. This a setup known as longitudinal dihedral, and allows aeroplanes to recover automatically from disturbances that knock them off course. Should a gust suddenly pitch your aeroplane nose-up, the change in angle means the wings produce a bit more lift and the tail a bit less downforce, leaving a restoring nose-down moment. Equally, if your aeroplane is bumped nose down, the wings produce a bit less lift, the tail a bit more downforce… leaving you with a nose-up restoring moment – just what you needed! Better still, this works passively without motors or controllers, and the pilot does not need to take any action to correct the flight path. Instead, the natural mechanics of see-saws and levers sort out the problem automatically.
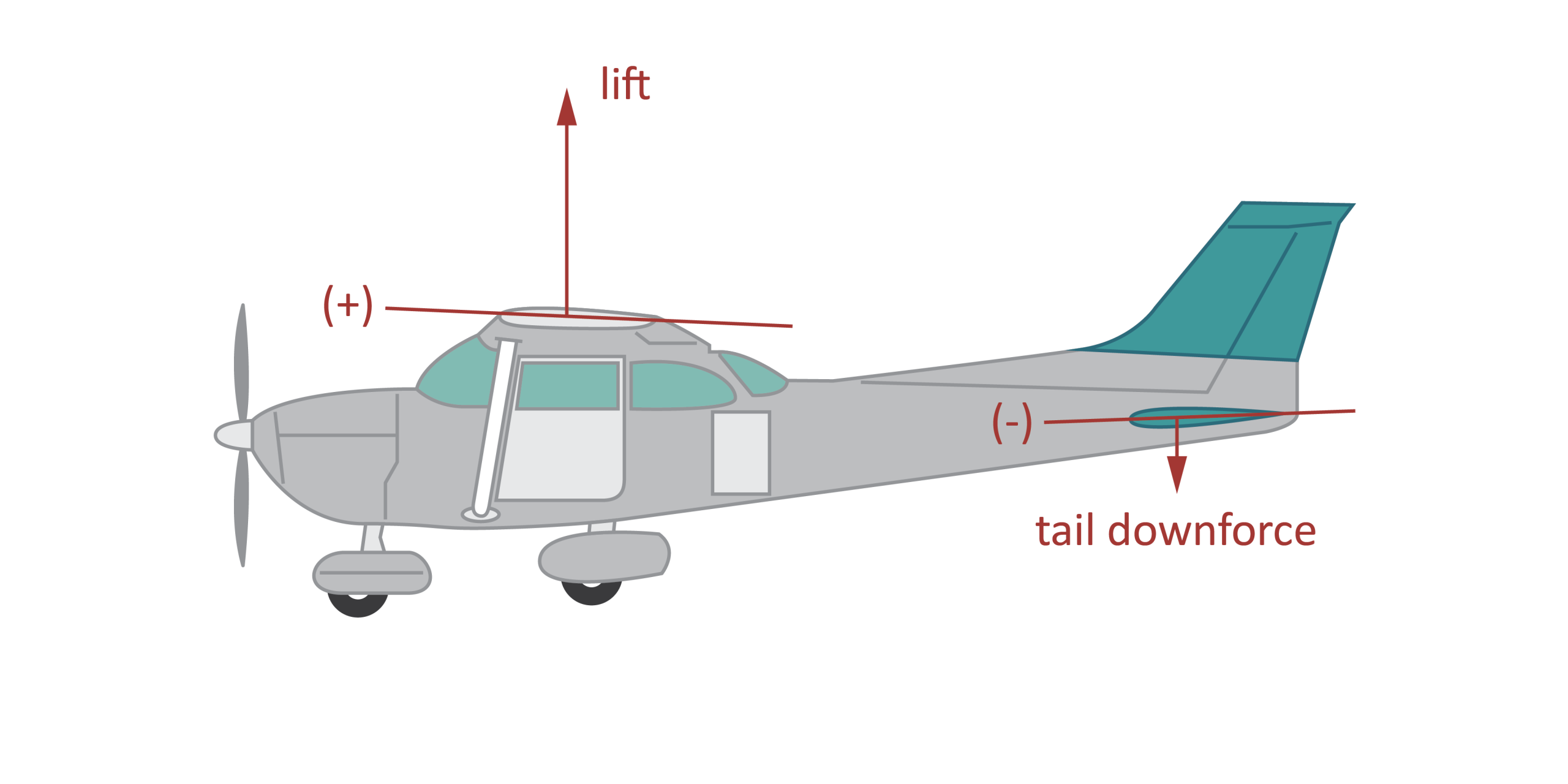
Most horizontal tails on aircraft produce downforce, so the wing must create more lift
Most horizontal tails on aircraft produce downforce, so the wing must create more lift
Birds use their tails in a different way
With our new knowledge of passive stability, we can return to birds. If birds have evolved the same design for stability as we have discovered for our aircraft, then we should see Lift generated by the wings and Downforce generated by the tail, right? That’s the type of hypothesis that we scientists love to test. All we need is a way to see and measure what the air is doing. Happily, our friends at LaVision have created a method of doing just that. Together, we filled the lab with soap bubbles containing a little added helium, so that they hung in the air. As the birds glided through this cloud of bubbles, they stirred them up, allowing us to measure their speed and direction and understand how the wake moves.
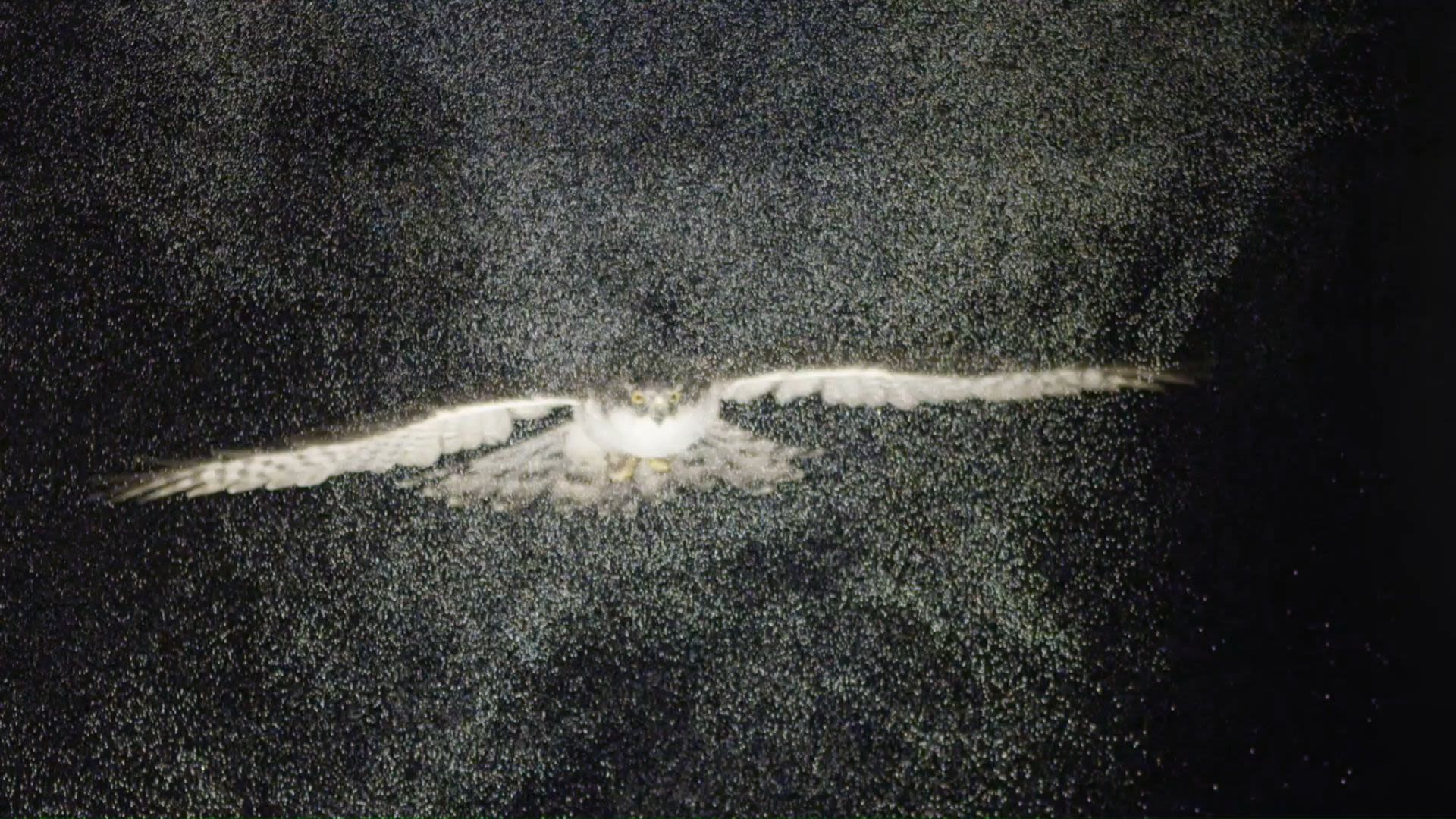
The wake is like a footprint in the air. It shows us what birds really do with their wings and tails
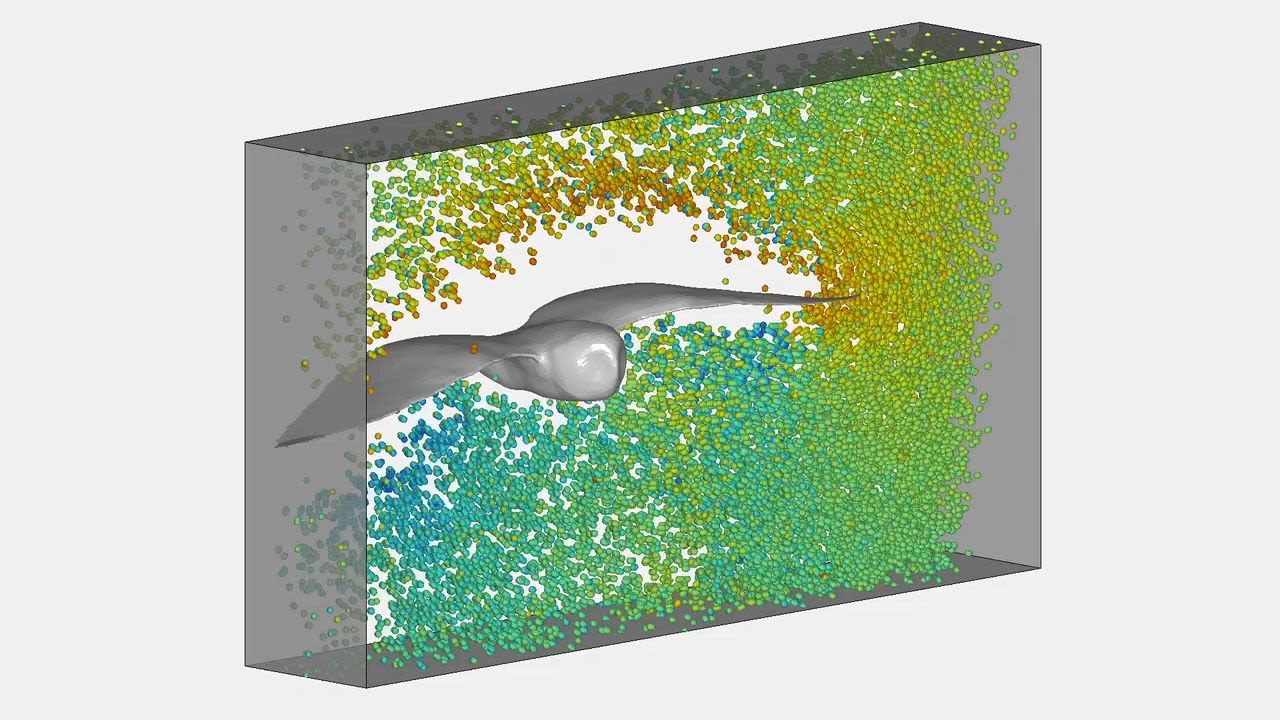
Efficiency over stability
With these visualisations, we see that birds operate quite differently from aeroplanes. Bird tails generate upward Lift, shown by the bubbles being pushed towards the ground. It turns out that they forego the passive stability we craved for decades when designing aircraft in favour of a passively unstable configuration. This means that birds must control their pitch angle actively, which takes some processing power, but there are twin advantages to this method. First, passive stability is a trade-off against manoeuvrability, so the birds will be more agile. When they want to pitch, turn or strike at prey they are not fighting against their own stabilisation mechanism. Second, they can greatly reduce their Drag, meaning flight is far more efficient.
Nowadays we have flight controllers that are fast, effective, cheap and lightweight. They appear in every quadcopter drone on the market. Like many others, we believe it is time to use active pitch control in the next generation of aircraft to save energy by increasing efficiency. There are already some large-scale aircraft, such as the Airbus A380 and assorted fighter jets, that generate Lift with their tails, but this trick is increasingly relevant to small-scale aircraft. This is because, for small things moving through the air, the fluid (yes – air is indeed a fluid!) seems relatively more viscous (not as viscous as honey, but moving in that direction on the stickiness scale). The component of Drag that birds are minimising is related to the air’s viscosity. This type of drag is comparatively minor for large aircraft because at larger scales the air is relatively less viscous.
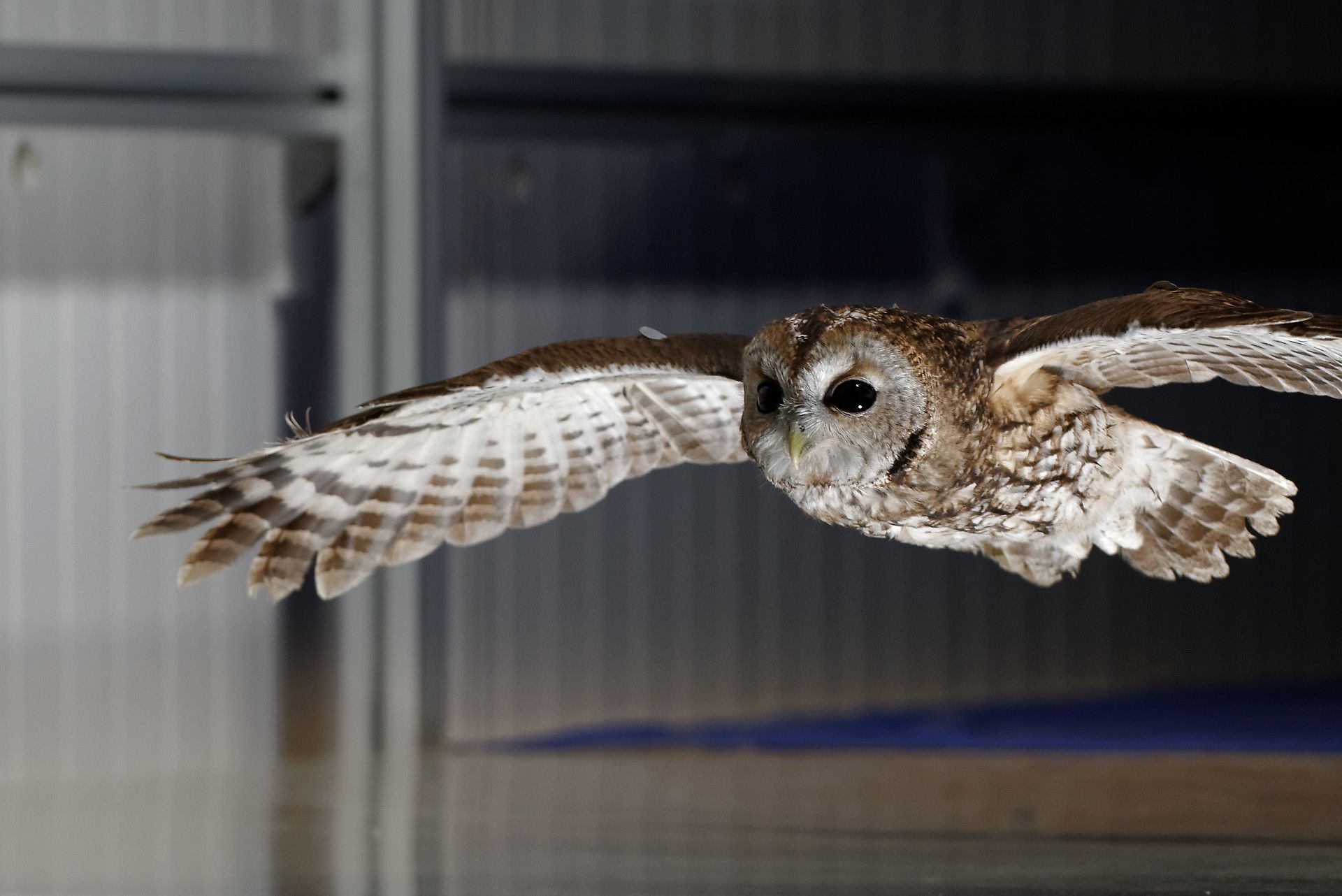
Johanna the tawny owl reduces drag by creating lift with her tail.
Johanna the tawny owl reduces drag by creating lift with her tail.
Lifting tails and hinging wings: these are just two examples of how the next generation of flying vehicles might embody improved engineering thanks to inspiration from nature.
In the future, quickly recognising the difference between a bird and a plane might be even harder than it is today. There are many other ways we might incorporate bioinspired design into modern machines or architecture – can you think of any?
Discover more
Discover more about our research with more amazing videos, instructions for making your own owl glider and many more resources.
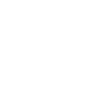
Digital content created by
RVC Structure and Motion lab
University of Bristol Research IT
Lloyd and Rose Buck – Bird Specialists
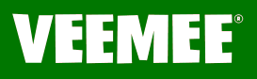